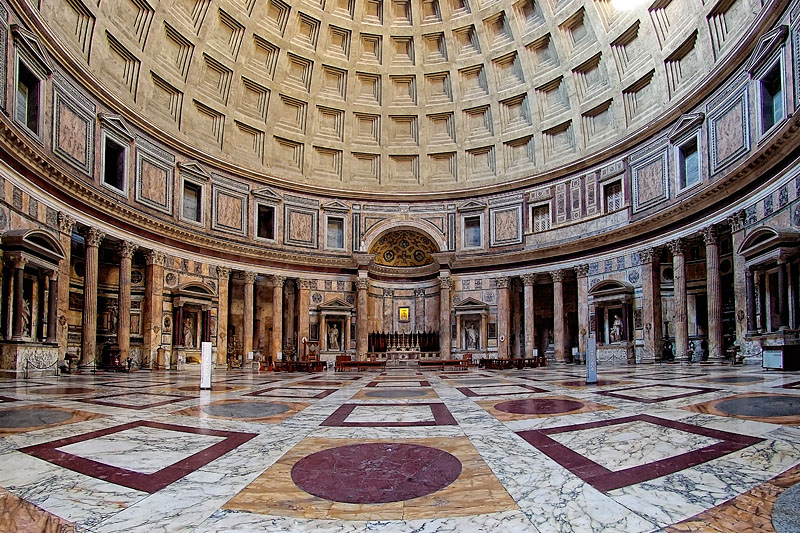
Concrete is an incredibly useful and versatile building material on which not only today’s societies, but also the ancient Roman Empire was built. To this day Roman concrete structures can be found in mundane locations such as harbors, but also the Pantheon in Rome, which to this day forms the largest unreinforced concrete dome in existence at 43.3 meters diameter, and is in excellent condition despite being being nearly 1,900 years old.
Even as the Roman Empire fell and receded into what became the Byzantine – also known as the Eastern Roman – Empire and the world around these last remnants of Roman architecture changed and changed again, all of these concrete structures remained despite knowledge of how to construct structures like them being lost to the ages. Perhaps the most astounding thing is that even today our concrete isn’t nearly as durable, despite modern inventions such as reinforcing with rebar.
Reverse-engineering ancient Roman concrete has for decades now been the source of intense study and debate, with a recent paper by Linda M. Seymour and colleagues adding an important clue to the puzzle. Could so-called ‘hot mixing’, with pockets of reactive lime clasts inside the cured concrete provide self-healing properties?
Concrete Recipes
At the core of modern concrete as well as mortar lies cement: this is the binder that is mixed with additional ingredients such as sand and gravel to turn it into concrete. Although each type of cement starts with calcium carbonate (CaCO3), the way this base material is processed and mixed determines how it cures, and what other material properties will be exhibited. The most basic type is called non-hydraulic cement, which starts off by firing the calcium carbonate (also called limestone), which produces calcium oxide and carbon dioxide:
CaCO3 → CaO + CO2
CaO is commonly called quicklime, as well as burnt lime. In the next step, this quicklime is mixed with water to ‘slake’ it:
CaO + H2O → Ca(OH)2
When the resulting calcium hydroxide is then exposed to carbon dioxide, the cement will begin to set:
Ca(OH)2 + CO2 → CaCO3 + H2O
At atmospheric concentrations of carbon dioxide, this is a very slow process and, thus, generally not very practical for construction. Common cement types such as Portland cement are, therefore, hydraulic cement, which sets by reacting with the clinker minerals that are part of the mixture. Portland cement is a fine powder which consists of of at least two-thirds of calcium silicates, primarily alite (Ca3SiO5) and belite (Ca2SiO4), along with additional silicates and aluminates.
The initial step of producing hydraulic cement is the same as for non-hydraulic cement, with the firing of calcium carbonate:
CaCO3 → CaO + CO2
The next step is where things get interesting, as the resulting calcium oxide reacts with the silicates and aluminates:
2 CaO + SiO2 → 2 CaO · SiO2
3 CaO + SiO2 → 3 CaO · SiO2
3 CaO + Al2O3 → CaO · Al2O3
Finally, the oxides (calcium oxide, aluminium oxide and ferric oxide from e.g., brownmillerite) react to form the final hydraulic cement mixture:
4CaO + Al2O3 + Fe2O3 → 4 CaO · Al2O3 · Fe2O3
At its core, this is the recipe which any hydraulic cement uses, whether it’s so-called ‘Roman cement‘, Portland cement, or Ancient Roman cement. When used to create concrete, this cement is mixed with aggregate, usually small rocks, stones, and sand. Upon adding water to this mixture, the hydration process will cause the cement to set without the addition of carbon dioxide.
Sands Of Time
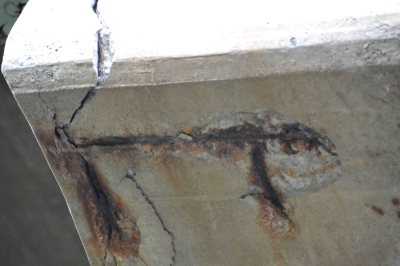
As with all building materials, concrete is also subject to many influences that degrade it over time. Some types of damage include mechanical and chemical sources, including oxide jacking and bulk fractures that chip away the material or causes cracks. Other types of damage are due to the metal rebar inserted to reinforce the concrete, with the corrosion of this rebar and subsequent volumetric expansion resulting in the concrete being destroyed from inside. Having cracks form in the concrete that allow moisture to seep in to reach not only the rebar, but also freeze during winters further work to limit the lifespan of modern concrete.
Preventing and repairing this type of damage has been the subject of much research the past decades, with a range of approaches attempted, including manual repair of visible cracks using cement, epoxy or resins. Even so, modern-day concrete is subject to rapid degradation due to the merciless effects of exposure to the effects of water. Considering the almost pristine condition of so much Ancient Roman concrete that survived over a thousand years of exposure to weather and the elements, this has obviously raised many questions.
Ancient Concrete
The ancient Romans were not the only ones who knew about and used concrete, with the ancient Greek also using the building material, even if not in quite the same amounts. One aspect about Roman concrete that we were already aware of was their use of pozzolan, specifically pozzolana such as volcanic ash. These are naturally rich silicates and aluminates, and a big reason behind the discovery of hydraulic cement by Roman engineers.
Since modern-day cements like Portland cement also include either natural or artificial pozzolans, their presence as ingredients can not be the sole reason. What other secrets have made it so that cracks and fractures haven’t shattered this ancient concrete into fine dust? For answers to this, the researchers of this recent study turned to a small former Roman settlement called Privernum in Italy.
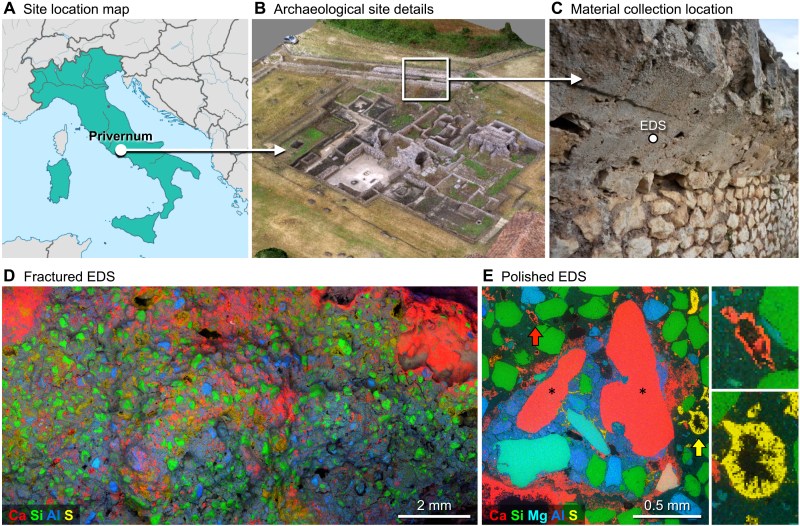
The Roman concrete test samples came from the archaeological site of Privernum, near Rome, Italy (A), and shown as a photogrammetry-based three-dimensional reconstruction (B). The architectural mortar samples were collected from the bordering concrete city wall (C). Large-area EDS mapping of a fracture surface (D) reveals the calcium-rich (red), aluminum-rich (blue), silicon-rich (green), and sulfur-rich (yellow) regions of the mortar. Further imaging of polished cross-sections (E) shows aggregate-scale relict lime clasts within the mortar (the large red features denoted by asterisks). The colored arrows in (E) denote the pore-bordering rings visible in the EDS data that are rich in calcium (red) or sulfur (yellow), which are enlarged at right to show additional detail. Photo credits (B and C): Roberto Scalesse and Gianfranco Quaranta, Associazione AREA3, Italy.
By taking samples of the concrete city wall of Privernum and studying its composition, they found that they saw pockets of calcium oxide, which was a feature that had been previously mostly dismissed as probably not relevant or just an artifact of the primitive methods used two-thousand years ago to make concrete. The theory that Linda M. Seymour and colleagues wanted to test here, however, was that these pockets of calcium oxide were not only there on purpose but that the engineers who created these mixtures likely knew what they were doing.
The Experiment
In order to test this theory, the researchers created batches of concrete inspired by their analysis of the Privernum samples, featuring Portland cement, pulverized fly ash, sand, and water. Across these batches, a varying amount of quicklime was added, and each batch cast into cylindrical molds. After curing for 28 days, the cured samples were compared with the Privernum samples, with the bright white of the lime clasts clearly visible in both. To test that it are these pockets of quicklime which enable the self-healing properties, the cured samples were split longitudinally and inserted into a flow circuit with 0.5 mm distance between the fractured sides of each sample.
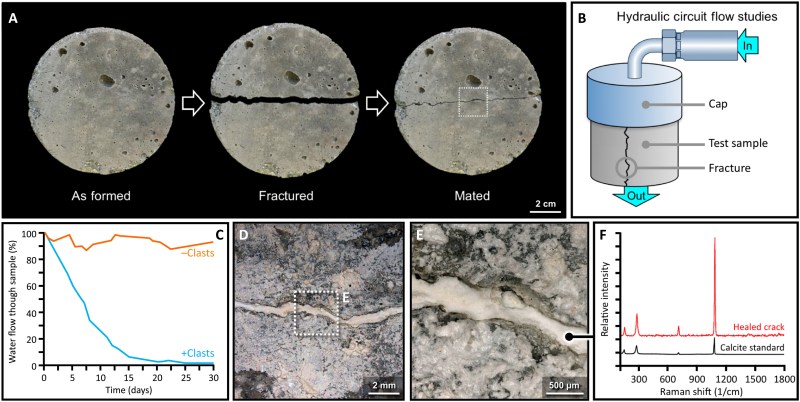
In this flow circuit, water was constantly forced through the fracture in each sample, with the flow rate measured. After an initial flow rate of 10 to 30 liters/hour, depending on the sample, after thirty days, the flow rate in the samples with the lime clasts was reduced to practically zero, whereas the flowrate in the regular concrete mix samples without the clasts had remained essentially unchanged.
The results of this experiment showed that the calcium from the lime clasts had likely interacted with the remaining free pozzolanic material to form fresh crystallization that is capable of healing fractures. These experimental results are indicated to match the analysis performed on the Roman mortars of the tomb of Caecilia Metella where a similar microcrack filling had been reported.
Vivat Romanorum Architectus
Despite so much of the engineering knowledge of Ancient Roman times having been lost over the past few thousand years, the gathered body of evidence on Ancient Roman concrete would seem to show unequivocally that self-healing concrete through the mixing in of coarse quicklime was the standard way in which such concrete was made. How this came to be, or which engineers first invented it will probably never be known, but it does appear to be a technique that can be applied to our modern-day concrete without question.
Linda Seymour and her colleagues considered this option and created a second mixture using coarse aggregates and a superplasticizer. Here the main concern was the shrinkage rate, which is mainly a factor of the setting process. The outcome here was that there was less than 1% difference between the drying shrinkage of regular and experimental concrete after a year, making it a potentially commercially viable concrete mix.
Whether we will see commercial concrete being poured using newly formulated cement mixtures based on this research remains an open question, but considering the benefits of having concrete structures that can outlast entire civilizations – both in terms of reducing the carbon dioxide output of the cement industry and a sharp reduction in the maintenance and replacement of concrete structures – it would be curious indeed if we did not.
(Heading image: “Inside the Pantheon” by Macrons on Wikimedia Commons)
Self-Healing Concrete: What Ancient Roman Concrete Can Teach Us
Source: Manila Flash Report
0 Comments